Lateral Lumbar Interbody Fusion and in Situ Screw Fixation for Rostral Adjacent Segment Stenosis of the Lumbar Spine
Article information
Abstract
Objective
The purpose of this study is to describe the detailed surgical technique and short-term clinical and radiological outcomes of lateral lumbar interbody fusion (LLIF) and in situ lateral screw fixation using a conventional minimally invasive screw fixation system (MISF) for revision surgery to treat rostral lumbar adjacent segment disease.
Methods
The medical and radiological records were retrospectively reviewed. The surgery was indicated in 10 consecutive patients with rostral adjacent segment stenosis and instability. After the insertion of the interbody cage, lateral screws were inserted into the cranial and caudal vertebra using the MISF through the same LLIF trajectory. The radiological and clinical outcomes were assessed preoperatively and at 1, 3, 6, and 12 months postoperatively.
Results
The median follow-up period was 13 months (range, 3–48 months). Transient sensory changes in the left anterior thigh occurred in 3 patients, and 1 patient experienced subjective weakness; however, these symptoms normalized within 1 week. Back and leg pain were significantly improved (p<0.05). In the radiological analysis, both the segmental angle at the operated segment and anterior disc height were significantly increased. At 6 months postoperatively, solid bony fusion was confirmed in 7 patients. Subsidence and mechanical failure did not occur in any patients.
Conclusion
This study demonstrates that LLIF and in situ lateral screw fixation may be an alternative surgical option for rostral lumbar adjacent segment disease.
INTRODUCTION
Spinal arthrodesis is a common surgical technique for degenerative lumbar spinal disease5,24,39). However, reoperation and fusion are sometimes necessary due to the progression of degeneration at a rostral or caudal segment adjacent to a previous spinal arthrodesis2,6,27,30,32). Typically, posterior decompression and/or fusion surgery are performed; however, reopening the previous operative site and dissecting through the scar tissue and around neural tissues are demanding surgical procedures7,11). By utilizing a retroperitoneal approach, those challenges may be obviated14). Indirect decompression and effective lumbar lordosis may be achieved with the insertion of a large interbody cage using a retroperitoneal route18,29). Currently, lateral lumbar interbody fusion (LLIF) is feasible due to improvements in surgical techniques and instruments14). However, the use of a stand-alone cage leads to subsidence and non-union in approximately 30% of patients1,23,34,37). Therefore, changes in the positioning and posterior instrumentation are necessary to prevent such complications. Moreover, the extension of the pedicle screw fixation requires the extension of the surgical field through the previous surgical site. The application of lateral screw fixation through the same LLIF trajectory may be an alternative to minimize the need for revision surgery25,31,36). We have utilized a minimally invasive screw fixation system (MISF) to perform lateral screw fixation after LLIF to treat rostral lumbar adjacent segment degeneration (ASD). The objectives of the present study were to describe the detailed surgical technique and the short-term clinical and radiological outcomes.
MATERIALS AND METHODS
Patient medical and radiological records were retrospectively reviewed. Surgery was indicated for patients with intractable back and leg pain aggravated by walking and improved by sitting/lying down, irrespective of the use of various non-surgical management for more than 6 months, due to adjacent segment stenosis and instability after a previous arthrodesis. All patients had undergone posterior single- or two-level arthrodesis from L3 to S1, and solid arthrodesis at the previously operated site was confirmed by computed tomography (CT) and dynamic plain lateral X-rays. Radiological instability was observed in all patients at the adjacent level (more than 10° of motion on flexion/extension radiographs or 4 mm of translation)22). Patients with osteoporosis and severe deformity according to the Scoliosis Research Society-Schwab Adult Spinal Deformity Classification, coronal deformity, >20° mismatch between pelvic incidence and lumbar lordosis, >9.5 cm sagittal vertical axis and >30° pelvic tilt were not considered for this surgery35). The surgery was performed in 10 consecutive patients from December 2012 to August 2016 (Table 1).
Clinical and radiological assessments
The clinical assessments were performed using patient-reported outcome questionnaires, including a Korean version of the Oswestry Disability Index (K-ODI,/45)21) and a visual analogue pain score for the back (/10) and leg (/10)16,18). All patients were encouraged to ambulate from the day of surgery using a lumbar brace, and patients were discharged on postoperative day 3. All patients were scheduled for follow-up visits at 1, 3, 6, and 12 months after the surgery and yearly, thereafter. The median follow-up period was 13 months (range, 3–48 months). Preoperative imaging included magnetic resonance (MR) imaging, a CT scan and plain radiographs (standing anterior-posterior, lateral neutral, lateral flexion, lateral extension view of the lumbar spine and anterior-posterior/lateral 36-inch whole spine radiographs). The same questionnaires were administered, and plain radiographs were obtained at each follow-up visit. A CT scan was performed at 6 months after the surgery and was repeated at 12 months postoperatively if solid bony fusion had not occurred.
The radiological analysis included pelvic parameters (pelvic incidence, pelvic tilt, sacral slope), the sagittal vertical axis (SVA) from the center of C7 to the posterior corner of S1, lumbar lordosis (LL) from T12 to S1, and thoracic kyphosis (TK) from T5 to T12 on the whole spine X-rays (Fig. 1A)17). The segmental angle (SA), anterior disc height (AH), and posterior disc height (PH) at the index level were measured using the lumbar X-rays (Fig. 1B). Solid fusion was defined as less than 5° of motion and 1 mm of translation on the dynamic X-rays and the presence of bony bridging on CT images4,5,9). Subsidence of the cage was defined as greater than a 2-mm encroachment of the interbody cage into the vertebral endplate on the plain X-ray images and/or CT scan. The measurements were performed using 150% magnified images, to ensure high measurement reliability33), and the tools of the picture archiving and communication system (Marosis, version 5483; Infinitt Healthcare, Seoul, Korea), which is compatible with Microsoft Windows (Microsoft Corp., Redmond, WA, USA)3,8). Two researchers who were blinded to the patient information performed each measurement, and inter-observer variability was assessed using intraclass correlation coefficients (ICCs). The Institutional Review Board (H 1608-093-735) approved our study.
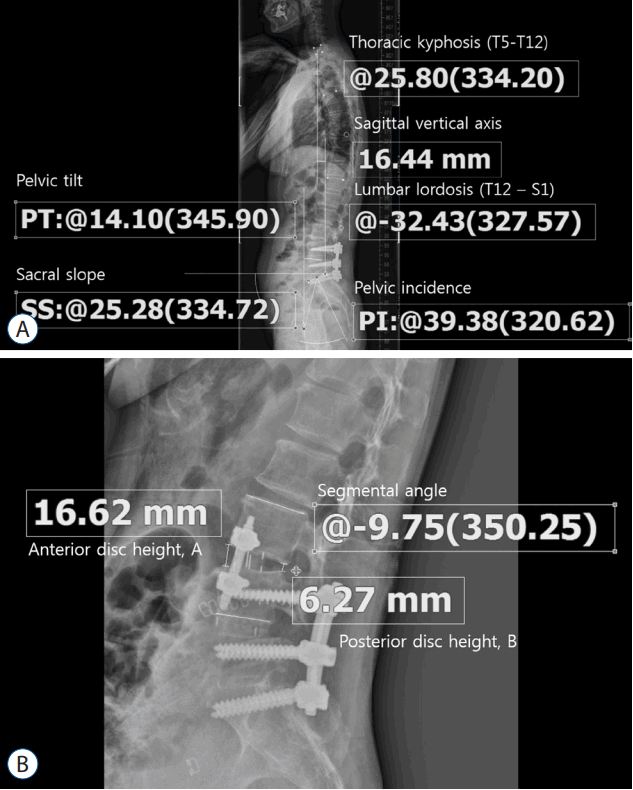
Radiological measurements. A: The sagittal vertical axis (SVA) is the horizontal distance from the C7 plumb line to the posterior-superior corner of S1. Lumbar curvature (LL) and thoracic kyphosis (TK) were measured between the superior endplate of T12 and S1 and between T5 and T12, respectively, via Cobb’s method using whole spine lateral radiographs. Pelvic parameters were measured using the measurement tools included in the picture archiving and communication system. B: The segmental angle (SA) was measured between the superior endplates of the fused segment via Cobb’s method. The anterior disc height (A) and the posterior disc height (B) on plain radiographs were measured to calculate the actual anterior (AH) and posterior disc height (PH). The length of the superior endplate of the L5 vertebra was measured on the plain radiographs (C) and computed tomography scans (C′). The actual length of the anterior disc and the posterior disc were calculated using the following formula: AH=A×(C′/C); PH=B×(C′/C). PI: pelvic incidence, SS: sacral slope, PT: pelvic tilt.
Surgical technique
The surgery was divided into two steps: interbody cage insertion and lateral screw fixation. The basic surgical technique and instruments have been described previously14,28). Under general anesthesia, the patient was placed in a true 90° right lateral decubitus position with a rolled towel under the right flank and secured in this position with tape. A modular table system (Mizuho OSI Modular Table System, Mizuho, CA, USA) was used, and the table was not flexed, similar to the oblique lumbar interbody fusion technique14). A 5-cm skin incision was made at the index level under fluoroscopic guidance. A probe was inserted at the anterior one-third of the disc space for stimulated electromyographic monitoring (NIM® X-PAK; Medtronic, Memphis, TN, USA). The probe was passed into the disc space through or anterior to the psoas muscle. After the confirmation of the location of the probe, serial dilators and tubular retractors were introduced. A discectomy was performed under direct vision using forceps, Cobb elevators and curettes. The size of the interbody cage was determined after the serial insertion of trial cages, and a permanent interbody cage that had been filled with allograft was implanted.
The second step consisted of the insertion of the lateral screws and rod through the same LLIF trajectory. MISFs were utilized to insert the lateral screws (Viper II®; Depuy Synthes, Arlington, CA, USA or Longitude®; Medtronic). The screw lengths were determined using the preoperative CT scans, and the screws were designed to reach the contralateral vertebral cortex. The tubular retractor was distracted, and a pilot hole with a tapper was made after an initial pilot hole had been constructed using a 16-G bone biopsy needle and guide wire (Fig. 2A, B) on one side. The segmental artery was coagulated and ligated under direct vision before creating the pilot hole, if necessary. The screws were inserted along the pilot hole via the MISF (Fig. 2C). In the other vertebra, the pilot hole was made as previously described or the hole produced by the stability pin was utilized. After the insertion of the guide wire through the hole, the screw was tapped and inserted. The rod insertion, application of the set screws, compression of the screws, and final securing of the set screws were sequentially performed via the percutaneous screw fixation system (Fig. 2D).

Surgical procedures. A: An intraoperative fluoroscopic image shows that the bone biopsy needle is inserted into the rostral vertebra, and a guide wire is inserted along the needle. The radiolucent tubular retractor shows that the procedure was performed along the same trajectory as the lateral lumbar interbody fusion. B: The pilot hole was constructed using a tapper along the guide wire. The second pilot hole in the caudal vertebra was made after the insertion of the guide wire through the hole created by the stability pin. C: Insertion of screws/rod. D: Assembly of set screws, compression of the screws and final tightening may be facilitated using a percutaneous pedicle screw fixation system. E: The screw was inserted from the left side in the anterior 1/3 of the vertebra, and the distal end reached the contralateral vertebral cortex. The screw was inserted anterior to the psoas muscle (white line) in this example. F: Solid bony fusion occurred at 6 months postoperatively. Note the location of the lateral screws.
Statistical analysis
Clinical parameters were compared between the preoperative values and those obtained at the last follow-up visit using the Wilcoxon signed-rank test. Radiological parameters were compared using a linear mixed-effect model, which represents correlations among repeated measures from a subject, and this model was used to assess the longitudinal changes in LL, TK, SVA, SA, AH, and PH during the 12-month postoperative period. The fixed effect was time, and the random effect was subjects. Post hoc analysis was not planned due to the small number of patients. Statistical analyses were performed using SPSS software for Windows (ver. 21.0; IBM Corp., Chicago, IL, USA). The results were considered statistically significant if p<0.05 (two-sided).
RESULTS
All patients were ambulatory upon discharge at 3 days after the surgery. The mean operation time was 81±14 minutes (range, 65–109 minutes), and the blood loss volume was less than 50 mL. The segmental artery was ligated intraoperatively in 2 patients. Transient sensory changes in the left anterior thigh occurred in 3 patients, and 1 patient experienced subjective weakness, all of which normalized within 1 week postoperatively. Back pain and leg pain were significantly improved after the operation from 8±2 and 7±2 to 5±3 and 4±2, respectively, on the VAS (p=0.04 and 0.02). The K-ODI decreased from 27±5 to 21±7 and showed a trend toward improvement (p=0.09). The ICCs showed satisfactory correlation (>0.9) for all radiological measurements except pH (0.72), and the mean values of each parameter were used in the present analysis. Two radiological parameters, SA and AH, changed significantly with time (p=0.002 and 0.011, respectively) according to the linear mixed-effect model, whereas the other factors did not show significant changes (p>0.05) (Fig. 3). SA and AH mostly changed during the first 3 postoperative months and stabilized thereafter (Fig. 3). Mechanical failure did not occur in any patients (Fig. 2E), and no cases of subsidence were observed. CT scans were obtained in 9 patients at 6 months postoperative, and solid bony fusion was confirmed in 7 patients (Fig. 2F). According to X-rays, 9 patients met the fusion criteria, without mechanical failure or subsidence. Those results may indicate sufficient stability of the lateral screws.

Changes in leg pain and radiological parameters. The graphs show that during the first 3 postoperative months, leg pain decreased, and the segmental angle (SA) and anterior disc height (AH) increased. Radiological changes were not evident thereafter. mo: month, VAS-L: visual analogue pain score for the leg.
DISCUSSION
The objectives of the present study were to describe the detailed surgical technique and determine the clinical and radiological outcomes utilizing LLIF and in situ screw fixation for rostral adjacent segment stenosis of the lumbar spine. The surgeries lasted approximately 80 minutes, and the patients had minimal bleeding. After the surgery, leg and back pain improved. The radiological findings showed that the SA and AH were significantly improved during the first 3 postoperative months without significant changes in the global spinal alignment.
Adjacent segment degeneration and surgical techniques
Due to the advancement of spinal instrumentation and surgical skills, the number of spinal fusion surgeries appears to be increasing10). The posterior fixation approach to lumbar fusion may increase stresses at the adjacent facet joint and promote degeneration, particularly at the rostral adjacent segment27). Symptomatic ASD, which is unresponsive to non-surgical treatment, requires reoperation. However, reoperation through a pre-existing surgical site is a demanding procedure. Although conventional revision surgery is performed posteriorly, it presents challenges such as a longer operative time, adhesions around the neural tissues and the risk of cerebrospinal fluid leakage7,13). Additionally, the extension of the rod to the newly inserted pedicle screw requires further opening of the previous surgical site7,11) and sometimes necessitates the opening of the entire previously operated site. Nonetheless, a strong advantage of conventional open surgery was the clear documentation of decompression under direct vision. Currently, direct decompression is possible with minimally invasive fusion surgeries such as transforaminal lumbar interbody fusion (TLIF), which is also another effective option38).
Currently, LLIF is feasible due to improvements in surgical techniques and instruments14). By circumventing the surgical route of the previous operation, the difficulties associated with scar tissue and adhesions may be avoided14,20,26,36). Effective restoration of disc height and lordosis may be obtained via the insertion of a large-sized cage using the retroperitoneal route, and indirect decompression through ligamentotaxis may be achieved12,14,26,36). Previous literature has shown that the dural sac was increased approximately 130–140% compared to the preoperative value after direct lateral interbody fusion (DLIF)12,14). However, the use of a stand-alone cage was associated with a high risk of subsidence and non-union in up to 30% of patients1,23,34,37). Therefore, the use of instrumentation to prevent subsidence may be necessary. Instrumentation may be applied posteriorly by connecting to the previous rods using rod connectors. However, this technique required changing the patient position and reopening the previous scar, leading to a prolonged operative time. The use of instrumentation in the same position through the same skin incision may be desirable. Thus, a lateral plate and screw system may be utilized36). In the present surgery, we utilized a conventional MISF. The conventional MISF is easy to use and familiar to many surgeons19). Moreover, rod insertion, the compression/distraction of screws and the anti-torque final tightening of the set screws are possible within a small operative field. Typically, disc height asymmetry between both sides exists after the insertion of the interbody cage, and the sequential compression of screws after rod placement may be necessary to obtain symmetric disc height on both sides and to secure the cage to the vertebral endplate.
However, the use of one lateral screw in each vertebral body is insufficient to control rotation in the sagittal plane, contrary to the use of pedicle screws, although lateral bending may be controlled25,31). The present study showed that the SA and AH increased during the first 3 postoperative months, indicating that rotation was not completely controlled, although such changes did not occur thereafter. CT scans were obtained in 9 patients at 6 months postoperative, and solid bony fusion was confirmed in 7 patients (Fig. 2F). According to plain radiographs, 9 patients met the fusion criteria without mechanical failure or subsidence. These results may indicate sufficient stability of the lateral screws. Nonetheless, comparative studies with conventional surgeries and other minimally invasive surgeries such as TLIF are required to show the efficacy of the present surgical technique.
Limitations
The present study had several limitations. First, the results were obtained from a small number of patients and cannot be generalized to all patients. Second, in the present study, postoperative MR images were not routinely obtained, and decompression was not radiologically proven. Previous literature has shown an effective increase in the spinal canal with DLIF, but those data were obtained after the initial operation12,14). However, in the case of ASD, severe degenerative changes such as severe spinal canal stenosis, disc space narrowing and ossification of the yellow ligament were frequently combined. Therefore, the effect of indirect decompression may be limited due to the characteristics of ASD. Without postoperative MR images, documentation of sufficient decompression was not possible, which is a major limitation of the present study. The present surgical technique may be applicable when the insertion of a large-sized cage is preferable due to ligamentotaxis. Third, to provide stability in every direction, three-column lumbar stabilization is necessary31). Although a limitation of motion was possible with the lateral screw system, a biomechanical study showed that the stability was lower than that achieved with a pedicle screw system25,31). Therefore, the present surgical technique may be applicable only for the treatment of select patients with minimal instability. Fourth, although no permanent complications occurred, chronic irritation of the psoas muscle and genitofemoral nerve by the screw may cause difficulties. Due to these limitations, we also suggest direct decompression using TLIF as a primary choice for ASD. Although, we did not recommend the present surgical technique as a primary option for ASD, the present study showed the feasibility of DLIF and lateral screws as a minimally invasive technique. Because the posterior spinal structure and pedicles were preserved, this surgical technique may not hamper further surgery. Use of DLIF and lateral screws for ASD may be considered when multiple degeneration is present in the other spinal levels, and further ASD and re-revision surgeries could be expected, due to a lack of trauma to the posterior spinal structures15). A long-term follow-up study with a larger number of patients is required.
CONCLUSION
This study demonstrates that minimally invasive LLIF and screw fixation may be an alternative revision surgery option to treat rostral lumbar ADS. A long-term follow-up study with a larger number of patients is required.
Acknowledgements
This study was supported by a grant from the Korea Health Technology R&D Project through the Korea Health Industry Development Institute (KHIDI), funded by the Ministry of Health & Welfare, Republic of Korea (HC15C1320). This study was also partially supported by Grant No. 0420163070 (2016-1063) from the Seoul National University Hospital. The co-author (CHK) is a consultant for Richard Wolf GmbH.